With rising energy prices and growing concern over global warming, will advances in solar and alternative energies enable the development of affordable and efficient energy solutions. Caltech research on the energy conversion process may offer some insight.
Energy conversion, ways of converting sunlight to electric and chemical energy, offers promising advancements to make solar and hydrogen energies more practical and affordable. On the forefront of energy conversion research, the framework of developing disruptive energy technologies, is The Lewis Group, that is part of the California Institute of Technology’s division of Chemical and Chemical Engineering. The Lewis Group is working in several research areas some geared towards to better understanding of energy capture, conversion of light into electrical and chemical energy, and energy storage. These research projects include photo-electrochemical, which focuses on the chemistry of semiconductors and materials, surface modification of semiconductors to improve electrical properties, and nanocrystalline titanium dioxide that could potentially lead to significantly lower cost for converting sunlight to electrical energy. The Lewis Group research projects are much more than improving photovoltaic devices; they are also exploring ways to convert sunlight into stored fuel energy.
While we are providing a brief and very oversimplified view of the solar energy research, it is important to understand the dynamics of energy conversion because the conversion process impacts the efficiency and the production cost of photovoltaic (PV) devices and fuels cells. The Lewis Group has an interesting PowerPoint presentation available for download providing an overview of energy from consequences of CO2 emissions to the latest in new technologies to improve alternative energies. Of particular interest are the historical efficiency trends for various materials used in crystalline and thin-film solar cells, energy conversion strategies of turning light to fuel and electric, and the cost/efficiency tradeoff in photovoltaic devices.
Cost/Efficiency Tradeoff
Photovoltaic devices are limited in their practical efficiencies governed by the thermodynamic limits and production costs that involve tradeoffs in materials, production processes, and PV device packaging. The Lewis Group provides a thorough illustration of the efficiency trends for various PV devices materials such as crystalline silicon used in semiconductors as well as the new approaches to thin film PV including amorphous silicon, cadmium telluride (CdTe), copper indium deselenide (CIS) and copper indium gallium deselenide materials (CIGS). These thin film material could offer substantial PV devices price reductions as a result of higher efficiency or lower production costs.
Figure 1 Cost/Efficiency Tradeoff
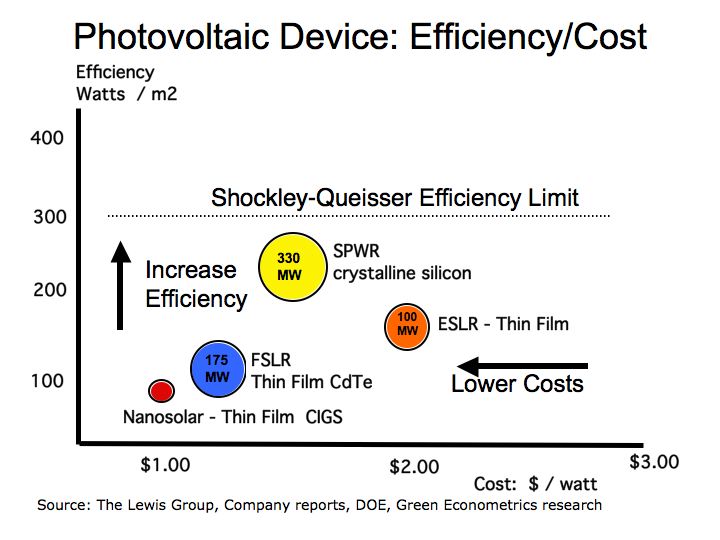
There is a tradeoff between improving the efficiency of a PV device, that is the amount of electric energy per solar panel, and the cost to produce the PV device. The average efficiency for a PV device is between 15%-to-16% and the average cost between $100-to-$350 a square meter. The cost per square meter and PV efficiency measured in watts per square meter can equate to a cost per watt, which ranges from $1.25/watt to $2.00/watt. SunPower (SPWR), offers the leading PV single junction device efficiency of 22% employing crystalline silicon and is expected to reach 500 megawatts (MW) production capacity by late 2009 from 330 MW in 2007. First Solar (FSLR) is ramping its thin film cadmium telluride (CdTe) solar technology by leveraging its low cost advantage with high volume production with its 75 MW in operations Ohio and 100 MW coming online in Germany. Startup Nanosolar is focusing on thin film solar PVs with a blend of copper indium gallium deselenide (CIGS) materials along with a manufacturing process much like printing, by placing the material blend upon metal foil. Evergreen Solar (ESLR) employs an integrated manufacturing process from wafers to cells and panels based on its proprietary String Ribbon Wafer technology with production levels at 118 MW in 2007.
There is presently a practical limit to solar efficiencies of approximately 30% and a thermodynamic limit of about 83%. The Shockley-Queisser limit at 30% for single junction solar cell efficiency is a function photon absorption in a material. “Detailed Balance Limit of Efficiency of p-n Junction Solar Cells” (W Shockley and HJ Queisser, Journal of Applied Physics, 1961) found that photons delivering excitation energy above a threshold (the band gap) for charge carrier electrons is lost to heating, meaning excess photon energy generates heat and not electric current. Photons with energy levels below the band gap pass through the material and photons above the threshold are absorbed. The absorbed photons transfer their energy to excite the electrons in the material and create pairs of negative electrons and positive charges that, because of their repulsion, travel to opposite ends thereby generating electric current.
According to the Lewis Group, other factors that limit PV cell efficiency include reflective loss of approximately10% for a material like silicon and a fill factor constraint that peaks at 83%. The fill factor restraint is attributable to the current-voltage characteristics of a PV cell that deals with matching the photocurrent density and voltage.
NREL (DOE lab) and Spectrolab (a Boeing company BA) using a multijunction semiconductor approach along with solar concentrators have achieved solar cell efficiencies of 40.7% in lab experiments while commercial products operate at 26.5%-to-28.3% efficiency range. Solar cell suppliers could reduce costs through production improvements or improve PV efficiencies without adding to costs. PV cell suppliers should benefit from economies of scale to reduce production costs while advances in technology improve PV efficiencies.
Research conducted by the Lewis Group at Caltech indicates that materials selected for PV devices have unique properties in their ability to absorb sunlight and generate electricity. In their analysis of semiconductor materials, the Lewis Group research found two parameters, determined by the physical properties of the material, that influence the PV’s efficiency. One is the thickness of the material, measured in microns, required to absorb enough sunlight to bring particular atoms in the material to an excited state, thereby freeing the electron to generate an electric current. The second parameter in the PV material is length of time the excited electrons last before recombining to generate heat instead of electric. Some materials such as silicon require material thickness of 100 microns while gallium arsenide (GaAs) only 1-to-3 microns.
Energy Conversion
Conversion of energy into electric or fuel is advancing through research in photoelectrochemical materials for solid and liquid fuels. Improvements in efficiencies for PV devices and fuel cells offer tremendous potential for transportation and home electric use.
Figure 2 Efficiency of Photovoltaic Devices
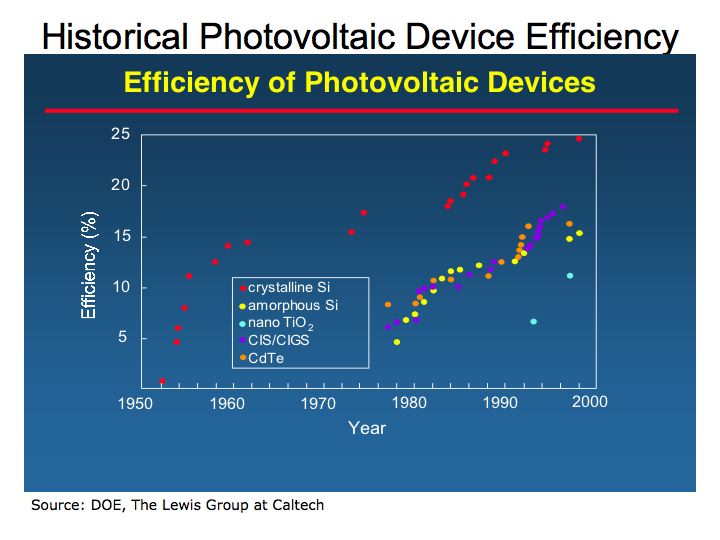
From figure 2, it seams quite apparent that PV efficiency has improved substantially since the 1950’s. Please see the Department of Energy (DOE) Basic Research Needs for Solar Energy Utilization for detailed analysis of solar energy. Further progress in PV and fuel cell technologies is predicated upon successful funding of further research. The bottom line is that only through innovative research will a truly disruptive technology be developed that has the ability of changing the economics to deliver an energy solutions to all nations.